2019
Experiment on Cavity Quantum Electrodynamics with cold Rb atoms. ― We set up a single mode optical cavity in the UHV chamber and frequency stabilized one of the fundamental modes (4 MHz linewidth) to the Rubidium 780 nm D2 transition line by piezo feedback and by means of the Ponder-Drever-Hall (PDH) method. We created a magneto-optical trap and Rb captured atoms from vapour. The fluorescence of the trapped atom cloud is shown in Fig. 1. We developed the absorption imaging technique to determine the number of atoms (~108) and the temperature in the trap, which, after polarisation gradient cooling, is about 100 microKelvin. The atoms, optically pumped to a well defined magnetic state, have been loaded into a purely magnetic trap which allows for transporting the atoms, eventually to position them into the volume of the optical cavity. The system is close to realise the atom-photon interface for the first set of planned experiments.
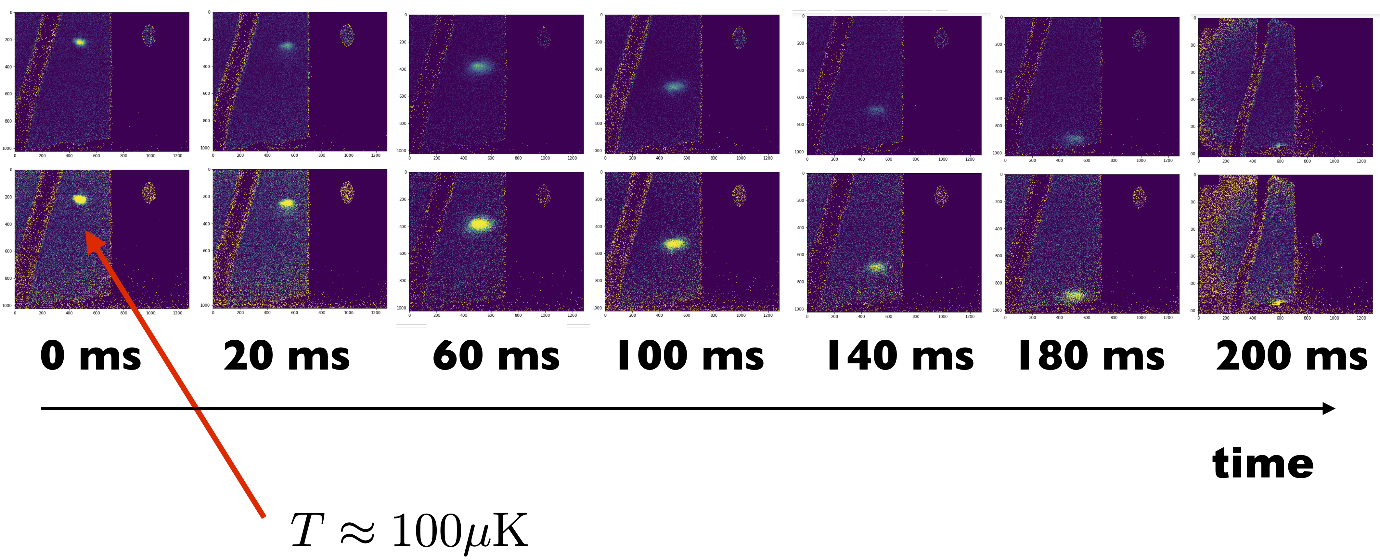
Figure 1. Adiabatic transport of cold Rb atoms in the magnetic trap into the optical cavity.
Cavity Quantum Electrodynamics, quantum critical phenomena. ― We completed and justified the interpretation of the photon-blockade-breakdown effect as a first-order dissipative quantum phase transition. To this end, we introduced the concepts of thermodynamic limit and finite-size scaling for the microscopic system of a driven dissipative Jaynes-Cummings model. The thermodynamic limit is defined for this microscopic system in such a way that the number of relevant degrees of freedom remains fixed. Instead of growing the system size, the scaling of the parameters keeps the form of the stationary solution of the driven-dissipative system invariant. At the same time, the proposed finite-size scaling leads to an increasing robustness of the attractor states associated with the bistability signal, until these states reach full stability in the thermodynamic limit. On approaching this limit, at variance with the fluorescence shelving experiment, no single quantum jump or other microscopic event can flip the system from one phase to the other. In the thermodynamic limit, the blinking telegraph-like signal vanishes completely and the state of the system is determined by the initial condition, similarly to the usual hysteresis behaviour in classical critical systems. If such a finite size scaling is possible – and here we show that this is the case for the photon-blockade breakdown effect –, then the bistability that can be observed in a given experimental realization of the system with its finite parameters not in the thermodynamic limit, can be considered the finite-size approximation of what is a genuine first-order phase transition in the thermodynamic limit.
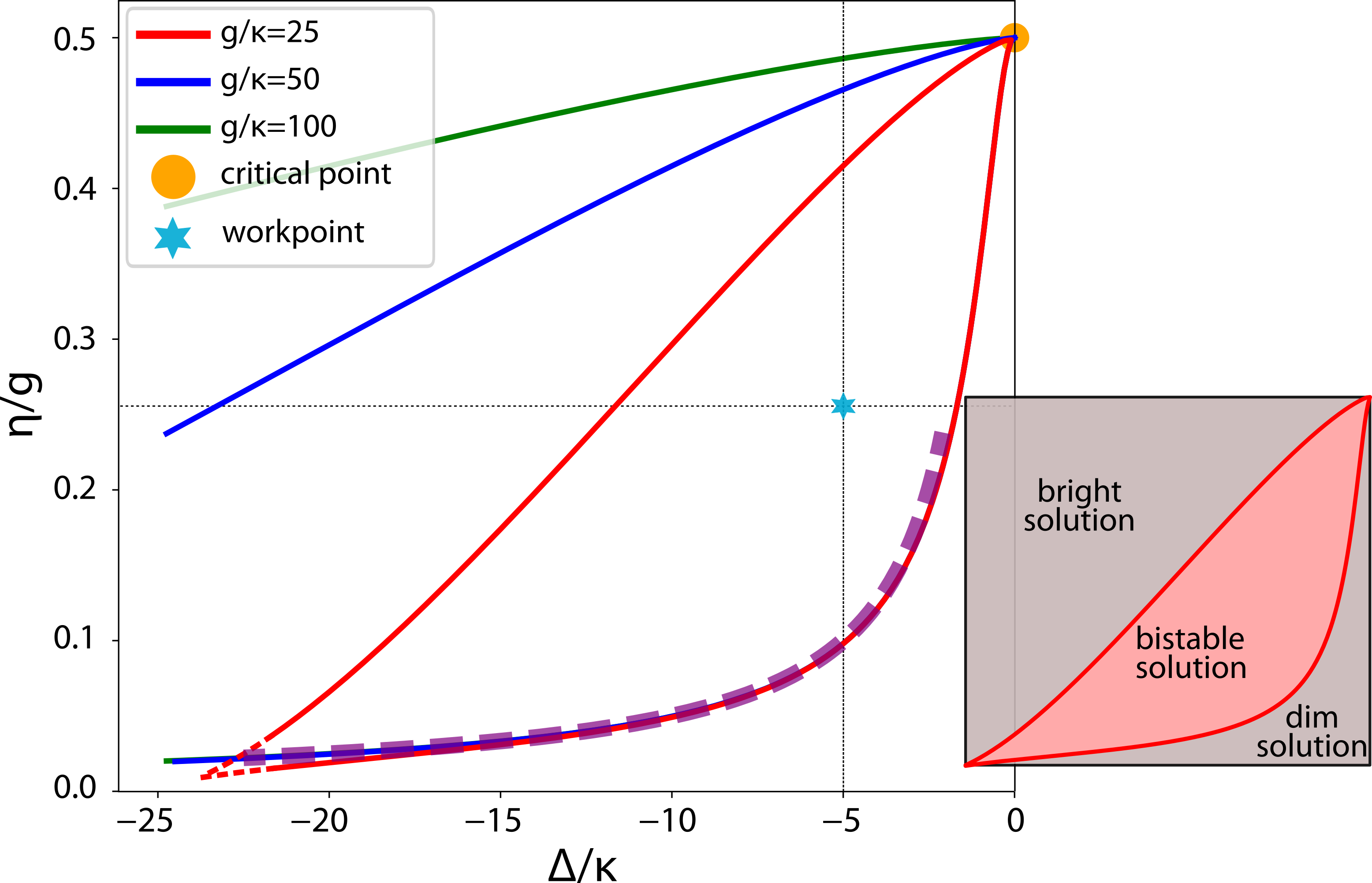
Figure 2. Classical phase diagram of the driven Jaynes-Cumings model in the ultrastrong coupling regime. The photon-blockade-breakdown phase transition can be induced by changing the drive amplitude (vertical axis) or the drive frequency (horizontal axis). We studied the finite-size scaling in the vicinity of the blue star, which is in the middle of the bistability region.
Numerical methods in Quantum Optics. — We developed a stepwise adaptive-timestep version of the Quantum Jump (Monte Carlo wave-function) algorithm. Our method has proved to remain robust even for problems where the integrating implementation of the Quantum Jump method is numerically problematic. The only specific parameter of our algorithm is the single a priory parameter of the Quantum Jump method, the maximal allowed total jump probability per timestep. We studied the convergence of ensembles of trajectories to the solution of the full master equation as a function of this parameter. This study is expected to pertain to any possible implementation of the Quantum Jump method.
External links:
[1] Vukics, Dombi, Fink, Domokos, Quantum 3, 150 (2019)
[2] Kornyik, Vukics, Computer Physics Communications 238, 88-101 (2019)